Electron transfer — the movement of an electron from one atom, ion or molecule to another — is a fundamental process in nature that underpins critical biological mechanisms like photosynthesis, cellular respiration and DNA repair.
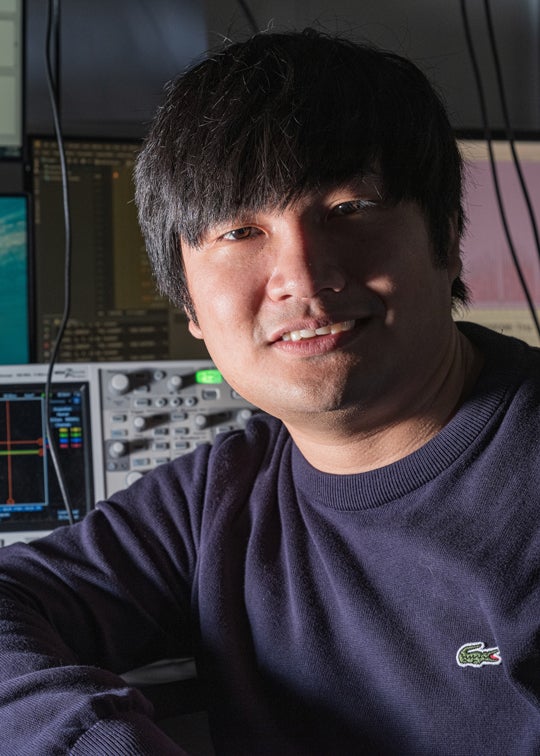
While the importance of electron transfer in these processes is well-established, the details of its mechanisms and rates remain elusive. Experiments alone cannot capture the full details, and computational models must include the entire system, not just donor and acceptor sites isolated from the environment, while also considering quantum mechanical effects. This makes real-time simulations of electron transfer dynamics very challenging with current computational power.
Physics graduate student Visal So, working in Guido Pagano’s lab, is addressing this challenge with an analog quantum simulator. Collaborating with Rice theorists José Onuchic, Han Pu and Peter Wolynes, Visal maps the molecular details of electron transfer systems onto the native light-ion interactions. This approach enables the creation of problem-specific, tunable simulators. “Trapped ions have an internal electronic level structure that allows you to encode and store quantum information,” Visal explained. “You can pick two levels that are very isolated and very clean for your qubit.” Using ion crystals trapped in an ultra-high vacuum system, the team has built a programmable quantum system that can independently control key factors in electron transfer models. These include donor-acceptor energy gaps, electronic and vibronic couplings, and environmental dissipation.
“Using our lasers, we have really precise control over the system,” Visal said. “We can tune the energy difference of these donor-acceptor systems. We also have control over the dissipation, so we basically engineer the environment for the system as well. This lets us study both the coherence of the system and the role of dissipation.”
The concepts of coherence and dissipation are central to the team’s work. Coherence, or an electron's ability to maintain a stable quantum state during transfer, follows quantum mechanical pathways of the electron's motion in the system. Dissipation, or the loss of energy into the surrounding environment, causes a loss of coherence and leads to motional equilibration. Together, they determine the electron transfer rate. And with the right amount of both, an optimal transfer rate can be achieved. By precisely controlling these parameters, Visal and his collaborators have successfully reproduced a popular model of molecular electron transfer on their quantum platform. These findings demonstrate the potential of their system to provide new insights into the dynamics of electron transfer.
Working with National Academy members Onuchic and Wolynes has been a highlight of Visal’s graduate experience. “Collaborating with these amazing researchers has given me confidence as a young researcher,” he shared. “It’s shown me that I can reach out to established researchers when I have ideas I’d like to pursue together.”
For Visal, the satisfaction of advancing our understanding of electron transfer is matched by the personal growth he’s experienced. As he continues his work, he remains focused on his goal of leading his own research group and contributing to the field of quantum simulation.