Illuminating a Cellular Mystery
Imagine looking into a cell and discovering a structure no one had seen before — something that had remained hidden despite decades of research. Peroxisomes, often overshadowed by well-known organelles like mitochondria and chloroplasts, were long thought to be simple sacs for enzymatic reactions. A breakthrough in Bonnie Bartel’s lab changed that perception entirely.
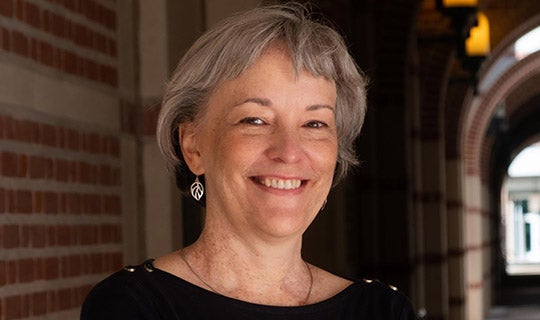
Bartel, Rice’s Ralph and Dorothy Looney Professor of Biosciences, didn’t start out studying peroxisomes. Her early research focused on auxin, a plant hormone that regulates growth and development in many ways, from vascular development to root branching and responses to light and gravity. As a geneticist, her approach was to disrupt biological processes to understand them.
“When geneticists want to understand something, we try to break it,” she said. “We look for mutants that can’t do the process that we’re interested in and figure out which genes are involved.” As she worked to “break” the auxin pathway, Bartel noticed something unexpected. Many of the mutant plants that produced less auxin also had defective peroxisomes. “We got a bunch of mutants that were defective in peroxisomes, and then I thought, ‘What’s a peroxisome?’” she recalled.
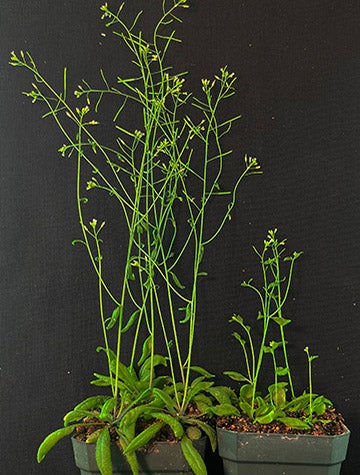
At the time, few scientists were studying peroxisomes in plants and even fewer were using genetics to investigate their function. That realization led Bartel to shift her lab’s focus, setting the stage for a major discovery.
Peroxisomes are found in nearly all eukaryotic cells — including yeasts, plants and animals — where they perform essential metabolic functions. Their name comes from one of their key roles: carrying out oxidation reactions that produce hydrogen peroxide. To prevent this byproduct from harming the cell, peroxisomes also contain enzymes that break it down into water and oxygen. Beyond this, peroxisomes help convert fatty acids into a usable energy source, a vital function for the cell.
In plants, peroxisomes have an additional critical job: supporting seed germination. This is especially important in oil-rich seeds like soybeans, peanuts and the Arabidopsis plants studied in Bartel’s lab. “Peroxisomes are especially important for plants during germination because seeds can’t do photosynthesis,” Bartel explained. “So, the mother plant packs the seeds full of nutrients, which can be proteins, oils or carbohydrates. If it’s oils, like it is for Arabidopsis, plants can’t access that energy unless they have peroxisomes.”
Bartel’s lab expanded its research to investigate peroxisomes from every angle. It studies how proteins find their way into peroxisomes from the cytosol, how peroxisomes form from endoplasmic reticulum and how damaged proteins within peroxisomes are identified and removed. The lab also explores what happens when peroxisomes themselves wear out — how they are broken down and recycled when they’re no longer needed. Another major focus is the interactions between peroxisomes and other organelles, especially lipid droplets, the place where cells store extra fats that are eventually metabolized in peroxisomes. “We’re interested in all things peroxisomes,” Bartel said.
As a first-year graduate student in Bartel’s lab, Zachary Wright ’21 was refining microscopy techniques to study peroxisome formation. He used bright, fluorescent reporters to highlight the membranes and internal spaces of the organelle, expecting to see the simple structure described in textbooks. However, when he finally began imaging in late 2016, the results were completely unexpected. “Everything looked totally different than it was supposed to,” Wright said. Instead of a uniform sac of enzymes, the peroxisomes were packed with intricate membrane structures. “It was like a bag full of all these tiny little vesicles,” he recalled.
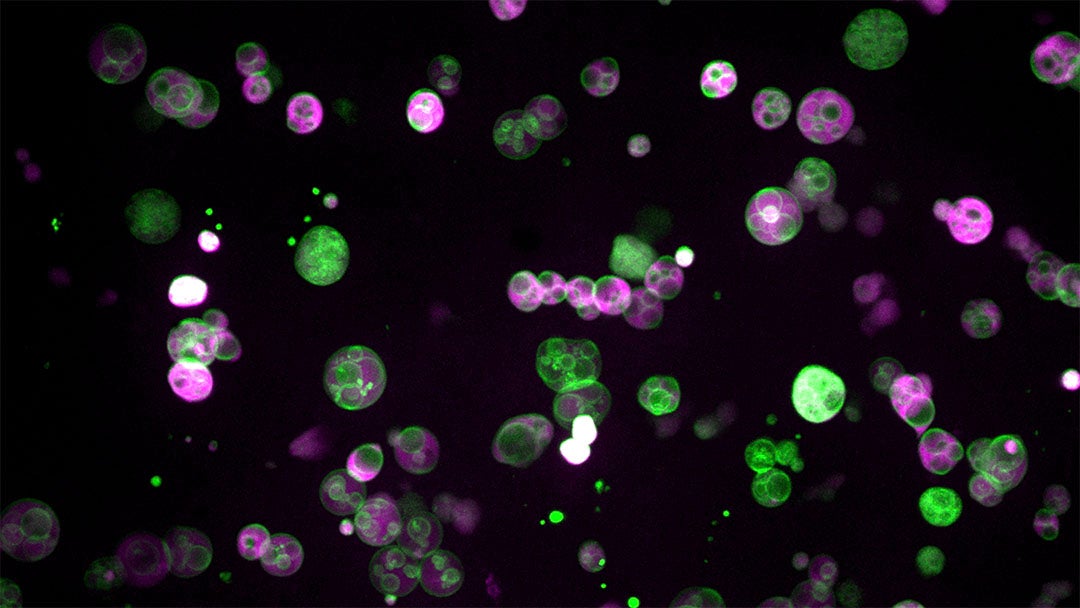
At first, it seemed impossible. “I thought it couldn’t be right,” Wright said. He suspected there was something wrong with the materials he had introduced into the plant cells. But when the same structure kept appearing everywhere, he began to reconsider.
“Then I showed it to Bonnie, and it was clear initially Bonnie didn’t believe it,” Wright recalled. “She was really nice about it. She didn’t just shoot me down, but it was very clear that she was quite skeptical of it, which is fair.”
They assumed the images must be the result of some artifact, something created by the experiment rather than a real feature inside the cell. Bartel admitted she had doubts. “I never thought Zach did anything wrong, but I didn’t think it was real,” she said. “If this was really happening, I thought somebody would have already noticed it.”
They kept working to convince themselves the finding was real. Wright checked his instruments, repeated his experiments and found no evidence that the structures were artifacts. He then turned to the scientific literature, searching for past references to similar internal compartments.

“I revisited the old literature about peroxisomes from the ’60s and saw they had observed similar things and just didn’t understand them,” he told Rice News in 2020. “And that idea was just lost.” Studies from the ’60s and early ’70s contained scattered references to internal compartments within peroxisomes, but in each case, researchers were focused on something else and mentioned the observations only in passing.
If peroxisomes in other organisms contained these vesicles, why hadn’t anyone explored them before? The answer lay in Arabidopsis seedlings.
Peroxisomes have been studied extensively in yeast and mammalian cells, but their tiny size makes it difficult to see their internal structure. Under a fluorescence microscope, they appear as nothing more than a dot because they are smaller than the resolution of light. In Arabidopsis seedlings, peroxisomes can grow up to 100 times larger — the size of an entire yeast cell.
“Zach followed up on the observation of a previous graduate student, Mauro Rinaldi ’16, who noticed that in very young seedlings, the peroxisomes are tiny, and then as they start using up the lipids, they get bigger,” Bartel said. “They noticed that there is a time in development when peroxisomes are naturally large, which was a breakthrough for us.”
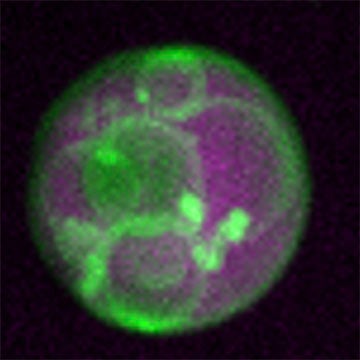
The newfound complexity within peroxisomes has opened new avenues of exploration for Bartel’s group. “Peroxisomes have more complexity than we originally thought,” she said. “These vesicles have something to do with how the peroxisomes are metabolizing fats, and they are very dynamic. We’re quite interested in understanding how they form, why they form and what they’re doing.
Bartel’s fascination with the unique properties of plants led her lab to focus on plant peroxisomes, but core peroxisome genes are highly conserved across plants, yeasts and animals, which suggests that the internal structures may be a fundamental feature of peroxisomes.
“Plants are innately interesting because they evolved multicellularity independently of animals, so they have a whole different way of being,” Bartel said. “But also, if you’re interested in basic cell biology, there’s a lot of advantages to studying plants. They’re easy to manipulate, and there are no ethical concerns like there are with vertebrates. We also have a unique advantage because plant peroxisomes are unusually large, and we can see inside them using light microscopy.”
She said her team’s discovery raises broad questions about peroxisomes’ role in cellular metabolism and offers a new perspective on human peroxisomal disorders. "This work could give us a way to understand some of the symptoms and potentially investigate the biochemistry causing them," she said.

A 2023 study from Bartel’s lab has already uncovered another surprising connection between plant and human peroxisomes. The team found that MIEL1, an Arabidopsis enzyme previously thought to function only in the nucleus, also localizes to peroxisomes in seedlings, where it helps break down lipid droplets for energy.
The discovery of a new role and location for MIEL1 prompted the team to question whether the findings would also apply to the enzyme’s human counterpart, PIRH2, a regulatory protein that cancers sometimes highjack to control a tumor-suppressing protein known as p53.
“PIRH2 is one of the most highly studied of p53 regulators, so it’s directly tied to cancer, because p53 is often mutated and implicated in a variety of cancers in a lot of different organs and cell types,” said Melissa Traver ’23, a former graduate student and postdoctoral researcher in Bartel’s lab. “Learning more about it could inform what we know about how cancer works and how to stop it.”
The Bartel lab’s discovery of dynamic vesicles within plant peroxisomes represents a significant milestone in our understanding of cellular biology — adding a new layer to our knowledge of peroxisomes and opening doors for future advances in science and medicine. Bartel’s work exemplifies how fundamental scientific discoveries lead to broader applications, underscoring the importance of foundational research in advancing science across disciplines.
“Being able to expose students to the importance of basic research and fundamental discoveries in science that are going to underpin the breakthroughs in science and engineering in the future is really important,” Bartel said. “We need to help convey the importance of basic research to our students and colleagues because that is something that has to happen in universities. There is no other place that can happen.”
— Sage Lee ’26 and Lauren Kapcha
Special thanks to Zoe Griffin ’25 for her contributions to the research and content for this article.
This work was made possible with support from the Fondren Fellows program.